7 April 2020–In complex fault zones, multiple seemingly disconnected faults can potentially rupture at once, increasing the chance of a large damaging earthquake. Recent earthquakes including the 1992 Landers, 1999 Hector Mine and 2019 Ridgecrest earthquakes in California, among others, ruptured in this way. But how can seismologists predict whether individual fault segments might be connected and rupture together during a seismic event?
One way might be to look for clues that the segments are connected below the surface, according to David Oglesby, a researcher at the University of California, Riverside. His study published in the Bulletin of the Seismological Society of America suggests that the pattern of slip distributions on fault segments can indicate whether segments separated by a gap at the surface are connected within a few kilometers of the earth’s surface.
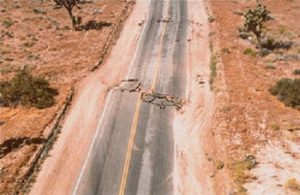
And in a second paper published in BSSA, Hui Wang of the Chinese Earthquake Administration and colleagues conclude that a rupture along a stepover fault, where parallel fault segments overlap in the direction of a rupture, might be able to “jump” over a wider gap between the fault segments than previously thought.
In both cases, making the connection between fault segments could have a significant impact on assessing seismic hazards for a region. “The potential maximum rupture length, hence the maximum magnitude [of an earthquake], is an important parameter for assessing seismic hazards,” said Mian Liu of the University of Missouri-Columbia, a co-author on the Wang study.
“The details of connectivity can have a controlling influence on whether you get a big earthquake that jumps across what appear to be multiple fault segments or a small earthquake that remains on a small segment,” Oglesby said.
Oglesby began thinking about this problem of discerning connections at depth after a conference where one of the speakers suggested that completely disconnected faults would have different slip patterns than faults connected at depth. Modeling that looked at slip distribution—broadly, where slip occurs along a fault—might be useful, he thought.
In his 3D dynamic rupture modeling of fault segments disconnected by gaps, Oglesby looked in particular at how rapidly the slip decays to zero at the edge of a fault segment on the surface. Does the amount of slip gradually decrease toward zero at the edge, or does it quickly decrease to zero?
The models suggest that “all things being equal, if a fault appears to be disconnected at the surface but is connected at relatively shallow depth, then typically the slip will decay very rapidly to zero at the edge of the fault segment,” Oglesby said.
Shallow depth in this case means that the segments are connected at about 1 to 2 kilometers (0.6 to 1.2 miles) below the surface, he noted. If the fault remains completely disconnected or is connected deeper than 1 to 2 kilometers, “then the slip will not decay to zero as rapidly at the edge of the surface fault segment,” Oglesby explained, since the deeper connection is too far away to have a strong effect on surface slip distribution.
Oglesby stressed that his models are simplified, and don’t account for other factors such as the high stress and strain and potential rock failure around the edges of fault segments. “And just because you get this rapid decay, it doesn’t necessarily mean that [a fault] is connected at depth,” he noted. “There are lots of factors that affect fault slip. It’s a clue, but not a smoking gun.”
In their modeling study, Wang and colleagues took a closer look at what factors might influence a rupture’s jump between parallel fault segments in a stepover system. They were prompted by events such as the 2016 magnitude 7.8 Kaikoura, New Zealand, earthquake, where rupture jumped between nearly parallel fault segments as much as 15 to 20 kilometers apart.
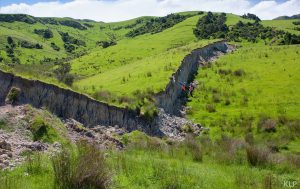
The researchers found that by including the background effects of changes in stress in a stepover, ruptures could jump over a wider space than the 5 kilometers (about 3.1 miles) predicted by some earlier studies.
Wang and colleagues’ models suggest instead that a rupture may jump more than 15 kilometers (9.3 miles) in a releasing or extensional stepover, or 7 kilometers (4.3 miles) in a restraining or compressive stepover fault.
Their models combine data on long-term tectonic stress changes with changes in stress predicted by fault dynamic rupture models, providing a fuller picture of stress changes along a fault over a timescale of both millions of years and a few seconds. “We realized that we needed to bridge these different fault models to better understand fault mechanics,” said Liu.
Liu also cautioned that their models only measure one aspect of complex fault geometry. “Although many factors could contribute to rupture propagation across stepovers, the step width is perhaps one of the easiest to measure, so hopefully our results would lead to more studies and a better understanding of complex fault systems.”